Glycated Serum Albumin Induces Interleukin-6 Expression in Vascular Smooth Muscle Cells
전체 글
수치
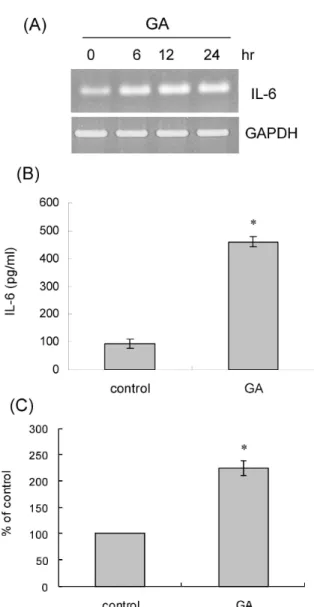
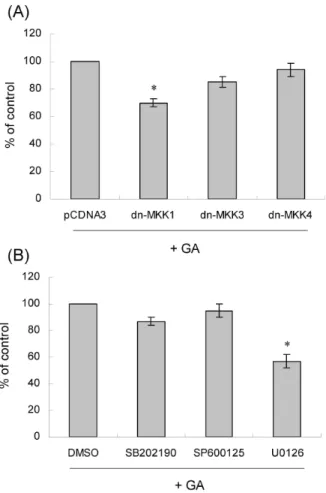
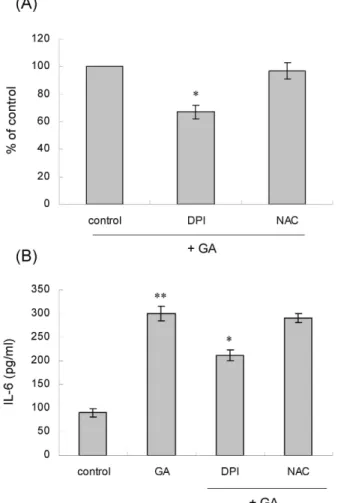
관련 문서
In the change of physical strength, The exercise group showed statistically significant differences in muscle strength(right), muscle strength(left),
We found that FoxO1 is constantly increased in MCF-7/ADR, adriamycin-resistant breast cancer cells, and FoxO1 has a critical role in the MDR1 gene expression (16).. There is
The combination treatment of PBA and 6-gingerol induced the cleavage of caspase-3, caspase-4, PARP, GRP78, p-eIF2α, and CHOP in DU145 cells and Western blot analysis for
Serum uric acid levels and risk for vascular disease in patients with metabolic syndrome... Prevalence if the metabolic syndrome in a Turkish
Conclusion: In conclusion, the relaxant effects of neuromuscular blockers on the uterine smooth muscle may be transmitted via nicotinic acetylcholine receptors
whether NME1-depleted cells impairs the Rad51 foci formation in response
포에서 We s t e r nbl ot 을 통해 HJ ME가 i NOS의 단백질 발현을 농도의존적으로 저해 하는 것을 확인하였다( Fi g.6) .그러므로 환삼덩굴은 i NOS의 발현을
To investigate whether oncogenic H-ras might modulate mdr1b expression in NIH3T3 cells, V12-ras-NIH3T3 and pcDNA3-NIH3T3 cells were transiently transfected with