Polyvilylidenefluoride-based Nanocomposite Films Induced-by Exfoliated Boron Nitride Nanosheets with Controlled Orientation
전체 글
수치
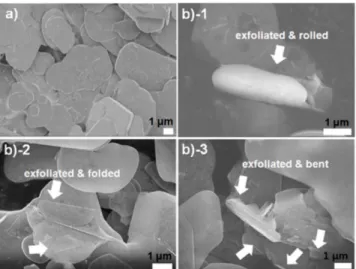
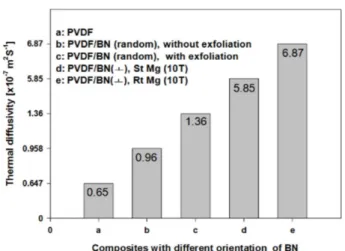
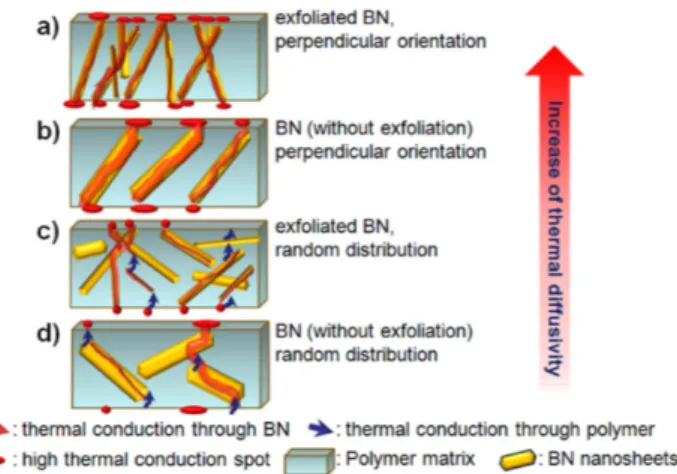
관련 문서
It considers the energy use of the different components that are involved in the distribution and viewing of video content: data centres and content delivery networks
After first field tests, we expect electric passenger drones or eVTOL aircraft (short for electric vertical take-off and landing) to start providing commercial mobility
If there are no results in each case, then your query result is identical to the answer query result, and therefore your query is correct (or at least, it is good enough that
1 John Owen, Justification by Faith Alone, in The Works of John Owen, ed. John Bolt, trans. Scott Clark, "Do This and Live: Christ's Active Obedience as the
Second, for the relationship between hospital capability and service orientation, the hospital capability had the positive significant impact on the service orientation in the
First, of human resources competencies in experience businesses, the customer service orientation and expertise besides achievement orientation had directly
Thermal balloon ablation versus endometrial resection for the treatment of abnormal uterine bleeding. Thermal balloon ablation in myoma-induced menorrhagia
4-15 The variation of flexural rigidity in accordance with graphite contents and roasting temperature.. 4-16 The variation of porosity in accordance