http://crossmark.crossref.org/dialog/?doi=10.14474/ptrs.2020.9.2.105&domain=pdf&date_stamp=2020-6-25
Effects of trunk control robot training on balance and gait abilities in persons with chronic stroke
전체 글
수치
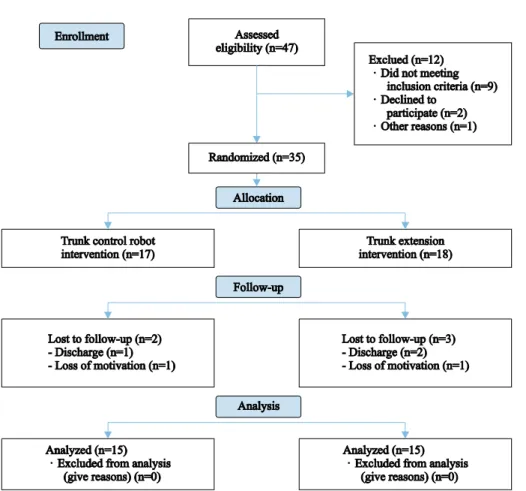
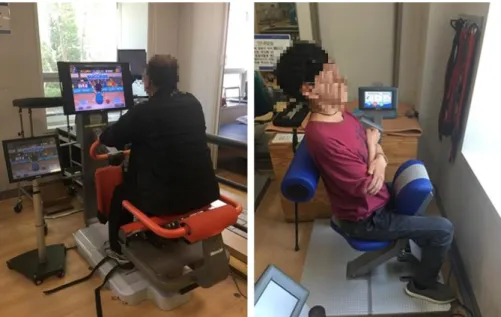
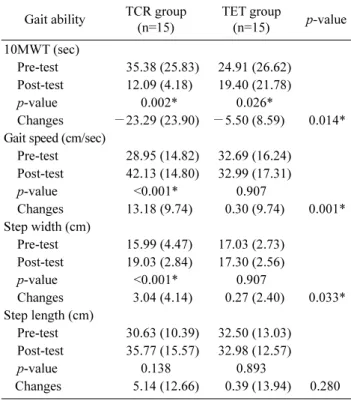
관련 문서
The aim of this study was to develop a training program for swallowing and to test its effect on swallowing capacity and nutritional status in stroke
In conclusion, the sling stabilization exercise program for middle-aged women with non-specific low back pain was found to be effective in improving
Therefore, nurses should be responsible for the relations to resolve the needs of care between nurses and patients and through communication with the patients,
Purpose: This study was performed to identify the association between family support, ADL and depression among hospitalized older patients with chronic
"Guidelines for the early management of adults with ischemic stroke: a guideline from the American Heart Association/American Stroke Association Stroke
period was prolonged unavoidably, (3) by explaining the risk factors associated with the failure to patients honestly, and subsequently performing
The torque analysis was performed acting on the joint of robot by modeling the kinematics and dynamics of the robot.. Load torque of the joint
• Apply the principle of impulse and momentum. Since the initial momenta is zero, the system of impulses must be equivalent to the final system of momenta. • Assume