Preparation and Characterization of Nafion Composite Membranes Containing 1-ethyl-3-methylimidazolium Tetracyanoborate
전체 글
수치
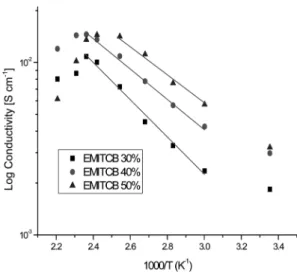
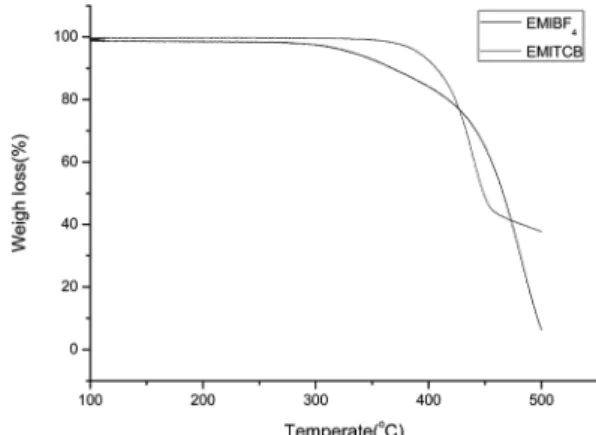
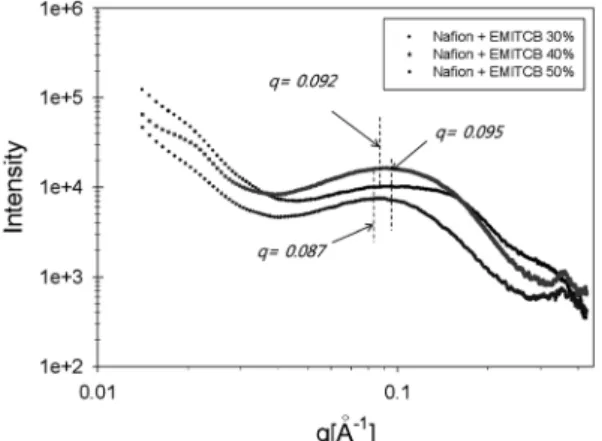
관련 문서
In other words, pomegranate extract has an effect of inhibiting the progression of alveolar bone loss due to periodontal inflammation by reducing the expression of COX-1 and
In an effort to develop a new method to remove nitrogen, this study examined the effects of C/N ratio, carbon source and nitrogen concentration on
Thus, an increase in circulation rate may decrease reboiler temperature, decreasing lean glycol concentration, and actually decrease the amount of water that is removed by
• An arrow with a negative sign (-): all else remaining equal, an increase (decrease) in the first variable decreases (increases) the second variable below (above) what
- Divergence: independent of (initial a.o.a) and airfoil camber the increase in aerodynamic moment about the elastic axis due to an arbitrary change in a.o.a is equal
• Specific heat (capacity): the measure of the heat energy required to increase the temperature of a unit mass of a required to increase the temperature of a unit mass of a
In this thesis, basic design process for an icebreaker is introduced, a reamers’ effects on resistance performance in open water is examined by model tests and a
– Established the fundamentals of high temperature fuel cells such as the molten carbonate and solid oxide fuel cell.. – In 1958, he demonstrated an alkali cell using a